12408:34701
Research on Elementary, Middle, and Secondary Earth Science Teacher Education (working with teachers and future teachers in all settings)
Authors: Heather Petcovic, Western Michigan University; Kim Cheek, University of North Florida; Robyn Dahl, Western Washington University; and Nancy Price Portland State University
Jump Down To: Grand Challenge 1 | Grand Challenge 2 | Grand Challenge 3
Introduction
With the release of the Framework for K-12 Science Education: Practices, Crosscutting Concepts, and Core Ideas in 2012 and the subsequent publication of the Next Generation Science Standards in 2013, many states have ramped up efforts to include earth and space science across the K-12 curriculum. Only four states (Virginia, Kentucky, Kansas, and Nebraska) currently require an earth and space science course for high school graduation, yet all but four states (Vermont, Arkansas, Arizona, and New Mexico) accept an earth and space science course as a science elective credit (Wilson, 2016). At the elementary level, earth and space science content is typically integrated with other science disciplines; beginning in the middle grades earth and space science may be a stand-alone subject area. On the bright side, many more children are now exposed to earth and space science in early grades, yet less than a third of high school graduates have taken an earth and space science course (Wilson, 2016). Clearly there is opportunity to increase participation of youth in K-12 earth and space science in public education.
Expansion of K-12 earth and space science as well as effective teaching require a highly prepared teacher workforce. Nationally, fewer college students are enrolling in teacher education programs, with a decline of 30% enrollment reported over the last five years (Barth et al., 2016). However, students entering teacher education programs now have stronger academic profiles (as measured by incoming SAT/ACT scores), and more entering students are completing their programs (Barth et al., 2016). Yet as many as a quarter to half of graduates of teacher preparation programs do not go into teaching (DeMonte, 2016). Retention of new teachers has also improved nationally, with 17-20% leaving the profession in the first four to five years - as opposed to older reports of nearly 50% leaving the profession within the first five years (Gray & Taie, 2015; Goldhaber, 2015; Brown, 2015). These same reports suggest that higher quality incoming teachers are retained in the profession at higher rates.
Amid this mixed news of national teacher preparation and retention, the earth sciences continue to have the least number of discipline-trained teachers of the sciences (Figure 1). Based on analysis of data provided by the National Science Foundation, Wilson (2016) estimates that 1% of elementary teachers and 3% of secondary teachers hold a degree in the geosciences. At present, we do not know what fraction of K-12 teachers have had coursework in earth and space science, and what the exact nature of this coursework entails.
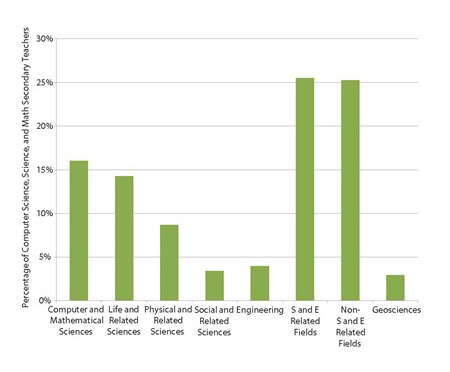
The charge of this working group was to consider what institutions of higher education can do to foster research on earth and space science teacher education. Early discussions among working group members quickly recognized that the K-12 teacher education landscape is influenced by a push-pull of many external factors such as higher education and K-12 institutional pressures and priorities, changing teacher education accreditation standards, high stakes testing, state-by-state NGSS adoption, and public perception of the value of earth science. Tackling this complex landscape would be well outside the scope of our small group of teacher educators, even with input from the broader earth and space science community. We chose to focus our grand challenges around three main themes that emerged from a longer list, and met the focus of the workshop as being within the sphere of undergraduate education research. These are:
- How do we attract and support a greater number of future K-12 earth and space science teachers who represent the diversity of K-12 learners?
- What are effective models for incorporating earth and space science into undergraduate K-12 teacher preparation?
- How do we best prepare future K-12 teachers to engage in earth and space science to promote "3-Dimensional" learning as described in A Framework for K-12 Science Education: Practices, Crosscutting Concepts, and Core Ideas?
Below we introduce the rationale for each challenge along with key researchable questions. We describe both short- and long-term strategies that could yield insight into each challenge and ultimately drive forward both knowledge and practice.
Proposed Grand Challenges and Strategies in K-12 Teacher Education
1. How do we attract and support a greater number of future K-12 earth and space science teachers who represent and can effectively engage diverse K-12 learners?
With less than 3% of secondary STEM teachers holding a geoscience degree (Wilson, 2016) we have a tremendous opportunity to grow the earth and space science teaching workforce. Yet growth of this workforce should reflect the growing diversity of K-12 learners, inclusive of gender, race/ethnicity, ability status, and more. Nationally, four out of five teachers are white, yet nearly 50% of school-age youth are ethnically diverse (AACTE, 2013). This issue is compounded in the geosciences, where less than 11% of bachelor's degrees in geoscience are conferred to students of African American, Hispanic/Latino, or Native American/Alaskan race or ethnicity (Wilson, 2016). Petcovic et al. (2016) found that only 2.5 % of institutions with geoscience departments were designed as minority-serving; as yet the fraction of teacher preparation programs in earth and space science at minority-serving institutions remains unknown. Research is needed to identify roadblocks that deter individuals, especially persons of color, from choosing or staying on the path to become earth and space science teachers. Research is also needed to identify mechanisms that are successful in both attracting individuals to K-12 earth and space science teaching and supporting their long-term success.
There is considerable overlap between this challenge and GC#2; here the focus is on understanding how to better attract and support the individuals who make up a diverse pool of future K-12 earth and space science teachers, whereas GC#2 focuses on broader institutional models, partnerships, and best practices in earth and space science teacher education.
Key researchable questions under this Grand Challenge include:
- How and when do individuals decide to become K-12 earth and space science teachers?
- What external factors contribute to or inhibit interest in becoming a K-12 earth and space science teacher, especially among persons of color?
- From where is the current and potential corps of K-12 earth and space science educators recruited, and how can we expand recruitment opportunities?
- How do we improve the transition from teacher preparation programs to the classroom in order to support diverse K-12 earth and space science teachers in their first few years?
Short term research strategies to address these questions include:
- At present, the research community lacks a baseline understanding of how individuals, especially persons of color, decide to become K-12 earth and space science teachers. We call for a systematic review of existing literature that would establish our current understanding of what attracts individuals to earth and space science teaching. This review should encompass literature in other STEM fields in order to establish what may be unique to earth and space science teaching in addition to what is common with other fields. It should also highlight critical theoretical and conceptual frameworks that provide explanatory power to findings.
- The research community also lacks a baseline understanding of what efforts in recruiting a diverse pool of students to K-12 earth and space science teaching have been successful. Again there is a need for systematic literature review that identifies the existing strategies for attracting students and determine what components of these are effective for underrepresented populations. We should look to other STEM fields for examples of successful interventions as well as to the results of programs specific to earth and space science teacher recruitment. Along these lines, there may be a need for comprehensive evaluation of NSF-funded GEOPATHS programs that focus on earth science teacher recruitment and preparation.
- To better understand the population of current and future K-12 earth and space science teachers, we suggest a survey of teacher preparation institutions that focuses on their recruitment methods. We especially would want to know how these institutions reach a diverse pool of potential applicants and the extent to which partnerships with two-year colleges and minority-serving institutions exist.
- The broader K-12 teacher education community has a good understanding of what factors support the transition from preservice teacher education to inservice teaching, inclusive of teachers of color (e.g., Ingersoll & May, 2011). However this work has not been communicated within the earth and space science teacher education community. We call for review and synthesis of this existing literature from which researchable questions specific to K-12 earth and space science teacher transitions may arise.
Long term research strategies to address these questions include:
- The geoscience education community has done some work examining awareness of and barriers to underrepresented populations pursuing study and careers within the geosciences (e.g., O'Connell and Holmes, 2011; Levine et al., 2007; Huntoon & Lane, 2007; Stokes et al., 2015; Baber et al., 2010; link to other references in the diversity GC group). To our knowledge, no work has yet been done to identify barriers and attractors to careers in K-12 earth and space science teaching. Building this understanding could take an ethnographic or phenomenological approach, drawing experiences from current K-12 earth and space science teachers of color to identify critical experiences (e.g., Levine et al., 2007). Initial work could be followed up with broader surveys of the current K-12 STEM teaching community to identify critical experiences, incidents, or factors that lead to greater interest in earth and space science teaching. Conversely, these surveys could also identify factors that drive interested students away from K-12 earth and space science teaching.
- Significant research supports the notion that reformed teaching practices lead to greater retention of STEM students, especially women and students of color (e.g., Freeman et al., 2014). Is the same true for future STEM teachers? To address this question, we call for a comparative study of whether institutions with transformed STEM course design might attract and support a more diverse pool of future K-12 earth and space science teachers than institutions with more traditional courses.
- We see a need for longitudinal phenomenological research that follows preservice earth and space science teachers into the first few years of teaching to identify factors that contribute to thriving. This is especially important for teachers of color, who are more likely to leave the profession within the first five years than are white teachers (Ingersoll & May, 2011). Current work points to organizational factors (such as the level of collaboration and autonomy, institutional support, and pressure of high stakes testing) as driving minority teachers from the profession (Ingersoll & May, 2011). Similar to the research agenda described above, we suggest an initial qualitative study followed by broad survey research to identify widespread factors that both contribute to K-12 earth and space science teacher retention, and those that ultimately drive teachers to leave.
2. What are effective models for incorporating earth and space science into undergraduate K-12 teacher preparation?
In order to produce K-12 teachers that are well-prepared to teach earth and space science, we must first determine what makes teacher preparation programs successful. Teacher preparation programs across the nation vary widely across several characteristics, including (but not limited to) content, course requirement, recruitment methods, graduation and placement rates, and student demographics. This is due in part to state- and district-level differences in teaching standards, and to differences in the types of institutions that offer teacher preparation programs. For example, most teacher preparation programs are offered at four-year universities but some are offered at two-year colleges. Other types of institutions, like museums and non-profits, offer components of teacher preparation like professional development workshops. A rigorous evaluation of teacher preparation must consider the diversity of contexts in which teacher preparation takes place.
Key researchable questions under this Grand Challenge include:
-
What pre-service and professional development teacher preparation models in earth and space science exist, and how do we measure the efficacy of these existing models?
-
How can models of teacher preparation expand beyond four-year institutions to reach two-year colleges, minority-serving institutions, and/or informal educational contexts (e.g., museums, science centers, nature centers, etc.)?
-
How can best practices for supporting special needs/underrepresented groups in earth and space science be incorporated into teacher education programs?
-
How do we prepare teachers to effectively integrate earth and space sciences with other STEM disciplines?
-
What are the current challenges within the domain for educators regarding pedagogical content knowledge (PCK), earth and space science content knowledge (CK), and science practices?
-
What institutional policies drive models of teacher education?
-
How do we prepare K-12 educators to teach earth and space science content and pedagogy in all settings (e.g., urban, rural, high needs, etc.)?
Short term research strategies to address these questions include:
-
There is a need to identify and evaluate existing models of teacher preparation that incorporate earth and space science, particularly those that specifically address the needs of elementary, middle, and/or high school pedagogical content knowledge (PCK). This could information could be obtained through a national survey, systematic literature review, or other mechanisms. Evaluation of existing programs should include methods for identifying their strengths and weaknesses.
-
While most teacher preparation programs exist at 4YC, some are hosted at other types of institutions, including 2YC, museums, non-profits, etc. These alternative pathways into teaching should also be identified and evaluated.
-
After existing models are identified and evaluated, additional research could be conducted to define the specific PCK, CK, and science practices needed by teachers of earth and space science at elementary, middle, and high school. This could be achieved through a literature review of research on earth and space science PCK and CK and would benefit from research on PCK in other science disciplines.
-
While earth and space science teacher preparation has unique characteristics, challenges, and opportunities, research on teacher preparation would benefit from collaboration with other science education organizations (e.g., NESTA, NAGT, ASTE, NSTA, NARST, etc.). Such a collaboration could also help teacher educators capitalize on the interdisciplinary aspects of earth and space science.
Long term research strategies to address these questions include:
-
Standards and other specific requirements vary widely from state to state and even from district to district within states. Successful teacher preparation can remain informed of local needs and ensure that teachers are fully prepared to enter the classroom by building robust partnerships with local districts. It is estimated that most teachers teach within 30 miles of where they grew up or went to college (Barth et al., 2016), so programs can be revised or designed with the knowledge that students enrolled in the program are very likely to teach in that region. Programs should be tailored to the unique needs and standards of local districts.
-
How is "success" defined for teacher preparation programs? We would argue that several metrics (e.g., recruitment of pre-service teachers into teacher preparation programs, retention, graduation rates, post-graduation employment, etc.) might be used in measuring the success of a teacher preparation program, and so measuring "success" is a complex endeavor. Furthermore, there are significant regional differences in teacher preparation requirements (e.g., state standards, district requirements, student populations, etc.), so direct comparison of programs is not always possible. We need to develop a methodology or tool for evaluating teacher preparation programs and models, so that we can determine and implement the most effective models.
-
The success of teacher preparation programs must include the long-term success of graduates after they leave the program and enter K-12 classrooms. Our evaluation of teacher preparation programs would benefit from the same type of longitudinal phenomenological research recommended above for GC#1. Successful teacher preparation programs should produce a K-12 teacher workforce that teaches well, reflects the demographics of the student population, experiences low rates of attrition, among other factors. This can be evaluated best through longitudinal studies of teachers as they move from teacher preparation programs into the workforce.
3. How do we best prepare future K-12 teachers to engage in earth and space science to promote "3-Dimensional" learning as described in A Framework for K-12 Science Education: Practices, Crosscutting Concepts, and Core Ideas?
The Next Generation Science Standards and A Framework for K-12 Science Education: Practices, Crosscutting Concepts, and Core Ideas upon which the NGSS are based reflect a new vision for K-12 teaching in science and engineering. Science is an interconnected enterprise encompassing three dimensions: science and engineering practices, crosscutting concepts, and disciplinary core ideas (National Research Council, 2012). To effectively teach earth science, K-12 teachers need to be understand the geoscience concepts they teach. They also need to be able to engage in the types of instructional practices that will help students progress in their learning of geoscience content over time (Duschl et al, 2011). However, K-12 science instruction as envisioned by the Framework is about more than teaching science content. There are important crosscutting concepts that cut across science disciplines (e.g., patterns, scale, stability and change, etc.). Geoscience teachers must understand how these crosscutting concepts apply in Earth and Space Science and be able to embed them in instruction. The Framework emphasizes that science learning occurs as students engage in the practices of science (e.g., making claims and arguments from evidence, developing and using models, etc.). Teachers must be able to engage in those practices themselves and be able to design instruction that will enable their students to develop facility with those practices. We know little at present about what effective three-dimensional teaching and learning looks like in geoscience education. There has been some research on students' use model-based reasoning in earth science (e.g. Rivet & Kastens, 2012), but literature that explores how students develop facility with other science and engineering practices in K-12 classrooms is lacking. Data on how students, teachers, or teacher candidates acquire crosscutting concepts is lacking.
Key researchable questions under this Grand Challenge include:
- How do struggles in teacher learning of earth science (e.g., misconceptions) impact instructional choices?
- What pedagogical content knowledge (PCK) is needed to teach effectively in the unique space of earth science - e.g., is there PCK for teaching in the field? for using big data? for visualizations?
- How do we integrate 3D learning into teacher preparation coursework and teacher professional development?
Short term research strategies to address these questions include:
- There is a need to identify teacher education instructional models that promote "three-dimensional thinking" in teachers, particularly as they relate to an understanding of "the nature of the Earth & Space Sciences." This is especially important as few K-12 teachers have strong backgrounds in geoscience (Wilson, 2016). A first step is a literature review to determine what teacher education models currently in use support three-dimensional learning, either specifically in the Earth and Space Sciences or in science education more broadly. There is some literature exploring practicing teachers' use of scientific argumentation (McNeill & Knight, 2013; Sampson & Blanchard, 2012), but we are not aware of studies that have investigated the development of teacher expertise with other science and engineering practices. We do not know how teachers acquire crosscutting concepts nor how to help them infuse these important themes into instruction. Once current teacher education models that promote three-dimensional thinking have been identified, we call for qualitative research that investigates specific teacher education models in the Earth and Space Sciences to determine their effectiveness in the promoting the nature of the Earth and Space Sciences.
- As NGSS-aligned assessments are developed, geoscience education researchers will need to conduct a literature review of available assessments for measuring the "three-dimensionality" of classroom instruction that could be applied to Earth & Space Sciences-specific instructional models. While many of these assessments are still in the early phases of development, research that evaluates the effectiveness of those models and their applicability for Earth & Space Science courses is called for.
Long term research strategies to address these questions include:
- K-12 student achievement in science is linked to the content and pedagogical content knowledge of their teachers (Jin et al, 2015). The Council for the Accreditation of Educator Preparation (CAEP) has standards for teacher preparation programs that focus on competencies that should be displayed by teachers of all subjects. A review of literature on the effectiveness of conceptual change instructional approaches in Earth and Space Sciences found far more research on astronomical phenomena than on geological ones (Mills et al, 2016). What is lacking is data that connects conceptual change instructional practices to three-dimensional learning. Research that measures the connection between teacher education instructional models that promote three-dimensional learning in the Earth & Space Sciences and the instructional practices K-12 teachers engage in in their classrooms will be important for both classroom teachers and geoscience teacher educators.
Conclusions and Connections
Many of the recommended research strategies require improved interaction with the broader K-12 teacher education community. Teacher education research within the geoscience education research community needs a greater presence in other teacher education organizations (e.g., Association of Science Teacher Educators, National Association for Research in Science Teaching, etc.) in order to address these Grand Challenges. Research in teacher education also must connect with practice; we need knowledge and models with practical results in order to better recruit, prepare, and retain K-12 earth and space science teachers who are capable of carrying out the vision of the NGSS Framework.
Grand Challenges within the area of teacher education connect to Grand Challenges as identified by other working groups. Many of the challenges in recruiting, preparing, and retaining a diverse K-12 earth and space science teacher workforce parallel issues of diversity and inclusion broadly in the geosciences (WG5). Reformed teaching practices at the undergraduate level may support the development of future teachers' pedagogical content knowledge, and help to support teacher recruitment and retention efforts (WGs 4 and 8). Future teachers struggle with the same cognitive, metacognitive, spatial, and other learning challenges as do other undergraduate students (WGs 1, 2, 6, 7, and 9). Research on professional development and faculty preparation in higher education parallels many of the same challenges and does research on teacher education (WG 10). In summary, research addressing Grand Challenges on many fronts will help to inform efforts directed toward K-12 teacher education.
References
Teacher Education -- Discussion
This is a very complex and challenging area of research, and the WG has been very thoughtful and thorough in the challenges that have been identified.
The training of elementary and secondary teachers is very different, and so are the licensure requirements. Typically a pre-service elementary teacher is required to take 9 credits of science content courses (and at some institutions 3 of these credits are earmarked for Earth and space science, but not everywhere) and 3 credits of science methods. A secondary science teacher needs to earn a BA/BS in the discipline. Given the different pathways, what prompts somebody to teach 4th grade children versus 9th grade Earth science differs significantly. Would it be possible to distinguish between the two groups, and identify the research challenges that are specific for each cohort, especially in GC#1? For example, surveying publications on the content and pedagogical approaches for Earth and space science courses for pre-service elementary teachers that have shown positive outcomes would be useful for the community.
12408:34809
A second aspect of the grand challenges you mention relates to the models of preparation, and raises the challenge of identifying ‘best teaching practices’ for supporting special needs children and underrepresented groups, as a need to identify specific PCK, CK and science practices. We have found much of the work on ‘ambitious teaching’ and ‘high-leverage’ teaching practices extremely helpful in this regard (Kloser, 2014; Stroupe, 2017; Windschitl, et al, 2012; Windschitl & Stroupe, 2017); and with these scholars we are finding a growing community of teacher educators who are working specifically in this arena. In our own program, as a next step in our research, we are aiming to develop a study that allows us to study how these ‘high leverage practices’ can be adapted for children in high-needs schools; for children who speak English as a second language; and for children with special needs.
Finally, in the grand challenges document you raise the challenge of defining ‘success’ in teacher education. As you note, there are a number of measures—recruitment of diverse candidates, retention, employment—(and we would also add retention in the type of school the program aims to prepare teachers) can help us understand and characterize whether a program is successful or not. In our own work we are examining many of the teacher-related outcomes you describe. We are also looking at student learning as one of the ways to measure the success of teacher education. This is, of course, a substantial challenge, and while there are many limitations to using an outcome measure such as the New York State Earth Science Regents; we also hope to begin to identify other more qualitative outcomes as well (which might include surveys of pupils in our graduates’ classrooms; samples of pupil work, etc.) that would provide a more elaborated understanding of our graduates’ impact on student learning. We would welcome colleagues who would be interested in thinking about and designing a means of looking at this important outcome with us as well.
We are so pleased to see that the NAGT GER Grand Challenges aligns so well with much of the work we are undertaking. We hope this public comment is useful in your work and thinking.
-Ro Kinzler and Karen Hammerness, American Museum of Natural History
12408:34827
I’ve noticed the important here and in other working groups themes (WG1 and WG2 esp) have placed on K-12 connections because of learning progressions and I think too because of research models. I also appreciate the comments that Cinza and Ro made about some of the fundamental logistics of how teacher prep programs are designed. So even though the focus of our Framework is on undergrad geoscience education research, we need to make sure our Framework is situated in the realities of how science education is done and that our teacher prep courses have external factors that drive decisions and can also drive the need for research questions at the undergrad level. Therefore I wonder if there is a way to make better use of the figures and create a figure that shows “the neighborhood” or “system” in which teacher prep at the undergrad level occurs. So in a conceptual way showing the connections to K-12 and to in-service, and to the fact that there are different state-national expectations/requirements, and that different elem ed vs middle ed, vs secondary teacher prep programs have very different design (e.g., housed in edu programs and geosci does some classes for them, houses in geo sci and student minor in ed, there is a a 5th year MS or no MS…). Maybe this is an impossible task, but if there is a way to show the main variables that teach prep programs are dealing with, maybe that will even help us “see” important directions for research. I think such a figure would also help support the important closing paragraph of your intro (just before you list the 3 GCs) where you talk about the push-pull external factors.
2. In intro regarding the statement that “1% of elementary teachers and 3% of secondary teachers hold a degree in the geosciences. “ and that “we do not know what fraction of K-12 teachers have had coursework in earth and space science, and what the exact nature of this coursework entails.”: While I think the 3% stat is powerful - I would want to see more HS teachers with geosci degrees, I do not expect elementary ed teachers to have a geoscience degree so 1% isn’t alarming to me. What is alarming and shocking is that the US doesn’t even know “what fraction of K-12 teachers have had coursework in earth and space science, and what the exact nature of this coursework entails.” To me this is among the most fundamental research needed in K-12 science education. If we can’t even report on the nature of the training, then how can we compare different types of training to determine which teacher prep program designs, course designs, experiences are most effective in the long term?
3. Intro: I really like that you had a clear statement of the charge for your working group. [“The charge of this working group was to consider what institutions of higher education can do to foster research on earth and space science teacher education.”] I think I’d like to see other working groups follow that model because it helps put clear but porous boundaries to the challenges and recommendations that your group proposed.
4. General comment: I like that you included suggestions for important researchable questions under each Grand Challenge, in addition to the recommended strategies. That wasn’t a requirement in the format, but I think it is very effective. I’d like to know if the others feel the same way, and if so then I would recommend that all WGs include a short list of important researchable questions under each of their GCs too.
5. Similarly, I like that you were able to break the recommendations into short term and long term strategies. But is the implication that the long-term strategies can’t be started until the short-term ones are researched/known? Or is it that the long-term strategies are larger-scale longitudinal studies that will simply (or not so simply) take more time than the short term strategies? Can you clarify?
6. I cheer on GC#2. I am currently teaching an Earth Science for pre-service teachers course (required for middle ed and an elective for elem ed), but was reluctant to teach again until I saw significant changes to address problems in institutional program coordination/overlap/rigor. It would be tremendously useful for me as an instructor and for the program overall to have comprehensive empirical evidence for best practices in program design and course design for the different populations we serve: elementary, middle and secondary pre-service teachers.
7. Do you get push-back, or do you anticipate push-bank on GC#4 because it focuses on NGSS (which I support) but state standards still reign in many states? I face this in VA – the pre-service teachers (undergrad and grad students) will be tested on VA SOLs not NGSS, so their motivation to learn NGSS is not so high.
12408:34842
I agree with Cinzia here, and would add a third category of middle school science teachers, who have yet different licensure requirements that are often even more variable. Middle school science teachers often have degrees in science teaching, but are still science "generalists." Middle school is where science interest and motivation in students starts to drop off and decline, so there are some interesting potential research questions for teacher preparation here that include motivating diverse learners in addition to integrating Earth and space science with other disciplines.
We developed some web pages through InTeGrate that might be helpful as references here, including a page that describes different types of teacher preparation programs, how they vary by institution type, and what the role of Earth science is (https://serc.carleton.edu/integrate/programs/teacherprep/teacherprep_whatis.html).
12408:35085
I, too, am a big fan of these kinds of systems diagrams. I started to pull together a lot of this information through InTeGrate, and some if it now lives in the pages about Preparing Future Teachers (https://serc.carleton.edu/integrate/programs/teacherprep/index.html). The reality is that teacher preparation programs are highly, highly variable.
There are some key references that are not included in this summary that will be helpful here:
- National Research Council. (2010). Preparing Teachers: Building Evidence for Sound Policy. Washington, DC: The National Academies Press. https://www.nap.edu/catalog/12882/preparing-teachers-building-evidence-for-so...
- National Research Council. (2015). Science Teachers' Learning: Enhancing Opportunities, Creating Supportive Contexts. Washington, D.C.: The National Academies Press. https://www.nap.edu/catalog/21836/science-teachers-learning-enhancing-opportu...
- Banilower, E. R., Smith, P. S., Weiss, I. R., Malzahn, K. A., Campbell, K. M., & Weis, A. M. (2013). Report of the 2012 national survey of science and mathematics education. Retrieved from Chapel Hill, NC: http://www.horizon-research.com/2012nssme/research-products/reports/technical...
- National Center for Education Statistics. (2016). Digest of Education Statistics, 2014. Retrieved from http://nces.ed.gov/programs/digest/d14/
- U.S. Department of Education, Office of Post-Secondary Education (2016). Preparing and Credentialing the Nation's Teachers: The Secretary's Tenth Report on Teacher Quality. Retrieved from Washington, D.C.: http://www2.ed.gov/about/reports/annual/teachprep/index.html
All of these reports provide more evidence about teacher preparation nationwide.
12408:35088
Introduction
Statistics and graphs from the AGI Workforce Report give a somewhat limited view of both teacher background and what students take. The National Survey of Science and Mathematics Education (Banilower et al., 2013) provides a wealth of detail that will be useful here. In fact, it answers one question posed in the introduction, about what fraction of K-12 teachers have had courses in Earth and space science. Some of what is in the survey, including the implications for teacher preparation, is summarized in Egger, A. E., Kastens, K. A., & Turrin, M. K. (2017). Sustainability, the Next Generation Science Standards, and the Education of Future Teachers. Journal of Geoscience Education, 65(2), 168-184. doi:10.5408/16-174.1.
Near the end of the first paragraph, there is a sentence that begins, "On the bright side...". I would be cautious about making judgments like that in a document like this one. In addition, what follows that phrase is a bit misleading. What is the current exposure to Earth and space science being compared to? How do we know that there is more exposure in the early grades now?
Grand challenge 1: Attracting and supporting teachers
This is a significant challenge that is not, of course, limited to the Earth and space sciences. One of the key aspects that seems to be missing in this description, however, is the demand for Earth and space science teachers. While we know that there are national, regional, and state-wide shortages in STEM teachers, how does that translate specifically to Earth science teachers? If ESS courses are not required, schools may not offer them, there's no demand for teachers. So some work to better understand the job market I think is important here.
One research strategy could be to survey current Earth and space science teachers and find out things like what other courses they teach, if they have or got other certifications, etc.
I'm confused by this statement: "Petcovic et al. (2016) found that only 2.5 % of institutions with geoscience departments were designed as minority-serving; as yet the fraction of teacher preparation programs in earth and space science at minority-serving institutions remains unknown." (First, I think "designed" is meant to be "designated"?) I think I understand the point being made, which is that our access to recruit broadly is limited by where there are currently degree programs, but I'm not sure that these statistics are really making that point well.
Grand challenge 2: Effective models
This is a very big challenge, too! But also a place where we are not starting from scratch. The two NRC reports I posted above are good general starting points.
I would move "defining success" up from long-term to short-term research strategies, because defining success is what will drive all of the rest of the research. Or, perhaps, you choose to not use or define "success" as a broad term and instead simply look for specific measures.
I think the idea of integrating Earth and space science with other disciplines is critical (given the job market issues described above), and looking for models of integration seems to me to be particularly timely.
Grand challenge 3: Three-dimensional learning
There will be a report coming out from the National Research Council in a few months that includes several chapters about this, so stay tuned!
The researchable question about misconceptions affecting instructional choices is fascinating, but I think is quite distinct from three-dimensional learning. There has been work on this in biology, I think, but not in Earth sciences so much.
12408:35091
The intro and GC1 are related to the need to address issues associated with extrinsic motivations for students in HS and college to earth science courses- in that some states (California and others), the role of Earth Sciences as the “easy” science is perpetuated by the university systems who don’t recognize those courses as college-prep (e.g. don’t count ES courses towards science requirements for college admission). Undergraduate students in pre-service teacher programs who can expect to only teach students who are not college-bound (or who didn’t take geosciences in high school because they were college bound) may not be as likely to enter geoscience teaching programs as those in chemistry, biology or physics.
GC2 could examine the efficacy of hands-on-teaching experiences such as the CSU, Chico “Hands On Lab” where they take a course to learn and practice teaching NGSS content to elementary students on class fieldtrips to the university.
12408:35388
While not specific to ESS teacher preparation, I would reinforce the prior comment on the NRC Document "Science Teachers' Learning: Enhancing Opportunities, Creating Supportive Contexts" as an important framing document. The original title of the committee was based on "Science Teacher Professional Continuum, which would support the overall aims of this working group.~
Consider also the growing literature on Teacher Induction Networks, especially given the requirements of the current NSF-Noyce requirements. There have been specific research requirements of late for these networks, and teacher induction plans are a prominent and required aspect of any Noyce funding at the present time.
I would strongly dissuade researchers from looking to CAEP for guidance, leadership or anything of use for framing research on teacher preparation. It is not CAEP's mission to collect any systematic data of value for understanding how to support more and better teachers of science. Given that less than half of the 31 states with whom CAEP has agreements on teacher accreditation even consider Specialist Professional Association (SPA) reports at the secondary science level, the impact of these standards is limited to between 10% and 20% of new science teacher certifications each year. These figures are based on a cross-reference analysis of Title II reports, NSTA's internal SPA data, and the more broad work of Richard Ingersoll. CAEP appears to be moving away from SPA reviews in any event, with the elementary standards SPA withdrawing and CAEP generating rather weak elementary content area standards.
NSTA in particular has been uncomfortable with the relationship with CAEP since 2013. This discomfort is based on a rather high cost for little return, particularly on the data front. As a result, NSTA and ASTE have been working to develop and implement revised teacher preparation recognition standards that are reflective of AFK12SE 3-Dimensional Learning, are comprehensive for K-2, 3-5, 6-8, and 9-12 grade bands. These new standards are designed to fill a role of program recognition as distinct and possibly separate from accreditation and provide a much more facilitative peer-review process. To the extent that NSTA and ASTE can determine, very little comprehensive data on the number and quality of science teachers in general has been collected. A data collection and research agenda can be tied to such data collection, and the ESS teacher preparation community is nimble and compact enough to take on a leadership role. Draft, non-public versions of revised standards would be available to the WG as they take into account comments in drafting a final version of this chapter. These are expected to be approved by the ASTE Board of Directors in June 2018 and the NSTA Board of Directors in July 2018.
I would also caution researchers to step back from NGSS slightly, given that many states are either non-adoption states, or "crypto-adopters." NSTA faced a bit if a push-back from state organization representatives from non-adoption states. Some state policy makers conflate NGSS with the Common Core, despite their separate and distinct natures. As a result, citing AFK12SE over NGSS has been a more productive, less "knee-jerk" reactive approach to policy on teacher preparation and professional development.
Finally, I would offer a meager and relatively narrowly known publication by Bonnie Brunkhorst and myself:
https://www.sensepublishers.com/media/1053-the-continuum-of-secondary-science...
Chapter 8 is based on Earth science teachers' needs, but the entire volume may be of use in the same manner as the NRC document.
12408:35406
Kim, your JRST citation is particularly relevant in the sense that science teacher preparation is a continuum as supported by the NRC report, "Science Teachers'Learning." Models are a central component of the work of teachers of Earth science, since they must, most likely, provide vicarious experiences about the Earth that defy simple laboratory-based examples.
12408:35409